[author: John Dulude]
Introduction
This paper follows up on a previous assessment and overview of energy system reliability challenges experienced during the February 2021 extreme cold weather event which affected the land area comprising the Electric Reliability Council of Texas (ERCOT) grid system. Winter Storm Uri significantly impacted the existing electrical grid, especially in Texas, serving as an important data point for assessing grid resilience and reliability. This discussion considers how the ongoing energy transition process may affect overall system reliability and how energy storage in its various forms may affect not only system resilience and reliability but costs to consumers, owners, shareholders, and those who manage risks, i.e., insurers and risk managers.
Electrical system grid resilience is the ability of a system to withstand adverse events and its ability to adapt to such events without suffering operational compromise. Simply put, resilience for an electrical system is its ability to withstand adverse events without sustained interruptions of service to customers. Resilience is largely about what does not happen to the grid or electric consumers. Reliability, on the other hand, is a measure of behavior once resilience is broken. The start of a sustained interruption is the transition point from the domain of resilience to the domain of reliability.[1] Some may argue with these definitions, and, in fact, some of the arguments may be valid, but for the sake of this paper we will consider these definitions for resilience and reliability as appropriate.
This paper looks at how energy storage at the grid-scale level may impact resilience and reliability, and how the current transition from a carbon-based to a non-carbon (or reduced carbon) system of generation within a grid may influence business risks. The following information may inform risk managers, insurance adjusters, and their legal support staff on the changing risks associated with energy storage during and after energy transition.
Objectives of Energy Transition
Before we can consider energy storage, its impacts on resilience and reliability, and how those impacts affect business risks and associated decision making, we must understand energy transition. Energy transition is the process by which all forms of energy take measures to achieve decarbonization, and one of the more important considerations while planning for decarbonization is determining the end goal. What is the measure? Is decarbonization achieved by carbon neutrality (balancing out the total amount of carbon emissions using offsets), or zero net carbon emissions (meaning net-zero carbon emissions into the atmosphere where a particular activity requires no offset?) Unfortunately, conversations regarding decarbonization goals and objectives tend to mix these terms. We can say with general agreement that energy transition is a process and not a result, but rather a means to an end.
Transition is “a change or shift from one state, subject, place, etc. to another” or “a period or phase in which such a change or shift is happening.”[2] In addition to addressing the overall objective of energy transition, we also need to define—within reason—how long the transition period will be, when it will be considered complete, how and where it will happen, and how will we know when it has been achieved. Globally, we are currently participating in a “phase” of transition, but we may not have fully recognized where that change will take our electrical energy delivery system. It is essential to keep in mind that energy transition is not just a change for the electrical grid but for all forms of energy. The scope of energy transition is an important concept to remember because different forms of energy are interrelated. For example, changes in the oil and gas sector will most definitely affect the electrical energy market. We are already seeing that effect on energy transition now through the market-based commodity system.
Energy Storage
AC, or alternating current, electricity is the operational format for electrical energy delivery in the U.S. from resources, such as the prime mover such as a turbine attached to a generator, to the load (customers). The electrical system in the U.S. operates at a frequency of 60 Hz, which means that the alternating current switches polarity 60 times per second. The reversal of polarity (positive and negative) with each cycle allows AC voltage to be more easily increased/decreased, which provides for more efficient transmission and distribution of energy from resources to the electrical load over long distances. Thus, our electrical system throughout most of the world is based on alternating current, and with such a system, supply and demand must be continually balanced in near real time. The ERCOT system almost collapsed in its entirety because of a system imbalance related to electrical frequency.
Because grid-scale electrical systems operate based on alternating current (AC), energy storage has typically been achieved by storing fuel prior to its transformation from mechanical energy to electrical energy by a prime mover, i.e., a turbine or drive system connected to a generator. Examples of those stored fuels include water behind a dam, coal in a pile, natural gas in pipeline, or fissionable material in a reactor. In today’s vernacular, energy storage has been more closely associated with grid-scale batteries of various types, which allow storage of energy from a fuel class (i.e., wind and solar) for future use in a form that can be quickly injected into the system as the need arises.
Note that battery storage systems utilize Direct Current (DC) and are interfaced with the AC grid through electronic inverter systems. DC electricity delivered by a battery maintains continuous polarity and does not alternate, as a battery has two poles or electrodes—a negative and a positive—and the current flows in a constant direction. A battery does not store electricity directly, but rather stores chemical energy produced by electricity, which it releases in the form of electricity by way of a reaction between the two electrodes of different chemical compositions.
In 2020, the U.S. had over 24 GW (24,000 MW) of energy storage capacity compared to 1,124 GW (1,124,000 MW) of total installed generation capacity.[3] Stored energy capacity represents just 2% of the total capacity in the U.S. system. Of that 2%, 96% exists in the form of hydroelectric pumped storage. Based on that figure alone, grid-scale batteries and all other forms of energy storage represent less than 0.1% of the U.S. generating capacity.
In 2019, Texas had a total summer capacity of 125,117 MW through all of its power plants[4] of which 20-25% of its current generation mix is in wind and solar. The capacity factor for wind which is defined as the resource availability both in terms of quantity and quality over a period of application is approximately 30% to 40% on average.[5] Capacity factor measures the overall utilization of a power-generation facility or fleet of generators.[6] From this relatively low capacity factor, it becomes apparent that anything short of hydroelectric pumped storage would not provide similar operational support over time for the carbon-based generation that it is either replacing or displacing. .)
This is not to say that intermittent renewables were at fault for the ERCOT system-wide failure during Winter Storm Uri in February 2021. Available data indicates that much of the lost generation in ERCOT was across all fuel types.[7] The consensus of many in-depth reviews of the historic extreme weather event in Texas indicates that market pricing inefficiencies and failure by regulators and owners to enact appropriate weather protection measures for fuel delivery contributed substantially to the breakdown.[8] [9]
Electrical energy is stored either chemically (Lithium-ion, sodium-ion, lead-acid batteries, etc.), electrically (capacitors, etc.), or mechanically (flywheels, pumped hydro, compressed air, etc.). Battery technology has seen a significant growth in deployment in recent years. According to some, the rationale is “renewables combined with battery storage are already an economically viable alternative to building new simple cycle gas turbine ‘peaker’ plants.”[10] Those holding to this rationale go on to explain that “pairing electricity generation with storage works especially well with solar energy, which generally follows a predictable daily pattern. In the U.S., costs have also been helped by the federal Investment Tax Credit, as high as a 30% tax rebate for new solar installations. Pricing and cost of battery storage has certainly come down and should continue to come down as technology improves.”[11]
Proponents of grid-scale battery storage assert that “the mass deployment of storage could overcome one of the biggest obstacles to renewable energy— its cycling between oversupply when the sun shines or the wind blows and shortage when the sun sets or the wind drops.”[12] Figure 1 compares many of the different energy storage technologies in terms of range of capacity (X-axis) and discharge time from least to most (Y-axis). Figure 2 compares various storage technologies and aligns them in terms of their range of capacity (X-axis) and installed capital cost (Y-axis).
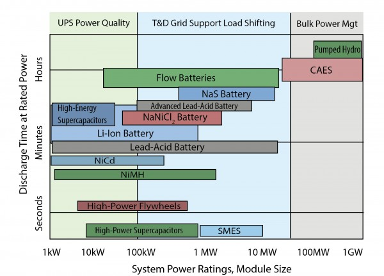
Figure 1 Characteristics/Positionings Of Energy Storage Technologies[13]
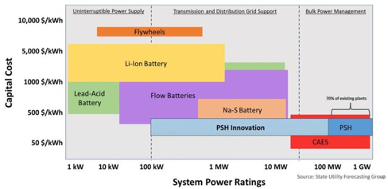
Figure 2 Capital cost and system power ratings of various energy storage technologies, highlighting the potential for m-PSH innovation (Welch, 2016, as adapted from the State Utility Forecasting Group)[14]
Despite the existence/contribution of net-zero and/or carbon neutral sources to available generating capacity, the current global electrical energy production is still 60% carbon-based, primarily comprised of coal, natural gas, and some oil. This ratio is similar throughout much of the U.S., even after 40 years of energy transition. In some parts of the world, carbon-based electrical generation exceeds 90% of the fuel mix. Professor Vaclav Smil, of the Faculty of Environment, Earth, and Resources at the University of Manitoba and a renowned expert on energy transition, stated, “Even a greatly accelerated shift toward renewables would not be able to relegate fossil fuels to minority contributors to the global energy supply anytime soon, certainly not by 2050.” The important takeaway from Professor Smil’s comment is that he considers our dependence on much of the products we use today, which are completely dependent on carbon-based fuels, as having more to do with a delay in energy transition rather than in the direct fuel application associated with electrical generation. His point is that even with significant inroads for converting the current carbon-based electrical generation system to one that is non-carbon-based, there are notable challenges associated with the manufacture of everyday goods that also depend on carbon-based fuels that will need to be addressed to achieve effective carbon neutrality or zero carbon emissions.
Policy makers and regulators generally, and certainly more recently, have mandated non-carbon-based fuel requirements with the expectation that this will hasten new developments in technology with an accompanying reduction in costs for renewable energy delivery systems. To a point, this has been the case; however, it has not fully avoided negative impacts to the reliability and resilience of the energy delivery systems. The challenge of transition continues to be the development of the correct mix of non-carbon-based generation that will have both the capacity as well as the duration to meet load requirements throughout various demand scenarios. Energy storage is the key to successful energy transition; however, it currently remains the weakest link in the energy transition process. Capacity challenges have in part been overcome more recently by reductions in cost per unit of energy delivered. The duration for which stored energy is available continues to be a major hurdle for energy storage. Except for hydro pumped storage, most forms of energy storage cannot economically achieve the duration requirements to support system reliability and resilience requirements. Until the increase in the duration component of energy storage can be better achieved at a reasonable cost, system reliability could be degraded during transition. Electrical service providers are finding themselves caught between mandates to reduce CO2 emissions and regulated operational requirements to meet specific delivery and service standards. Overcoming the energy storage dilemma will likely be key to unlocking the final phases of the energy transition process.
System Reliability and Resilience
Impacts to electrical system reliability and resilience may adversely affect both business continuity and economic risks. Change in risk means a change in risk management costs for businesses and for those who support the businesses, including customers, shareholders, and those that insure or underwrite business operations. In February 2021, Winter Storm Uri significantly impacted the existing electrical grid, especially in Texas, serving as an important data point for assessing grid resilience and reliability. Because it is such a significant and recent event, this paper will consider how energy storage may or may not have affected system performance. Significant impacts associated with Winter Storm Uri were realized on business operations primarily associated with business interruptions.
In the case of Uri, available data appear to indicate that the interruptions to consumers were part of a larger system emergency to meet electrical load demand which resulted in forced outages of electrical service throughout much of the state of Texas and beyond. It should be noted that there is no specific evidence that energy storage or the lack thereof played a crucial part in this shortfall or that it could have completely prevented the outcome. It is worth noting that generation reserve margins prior to the storm were significantly overestimated and the lack of available reserve, whether in the form of standby reserve generation or energy storage resources, could have significantly reduced the eventual impact of that storm.
A preliminary finding from a joint report by the Federal Energy Regulatory Commission (“FERC”) and the North American Electric Reliability Corporation (NERC) “highlighted the rapid need for more battery storage to support the state's grid. Preliminary findings of a joint investigation by the FERC and NERC blamed an increasing frequency of extreme cold weather events, as well as the devastation caused by the failure of natural gas-fired plants.”[15] While the lack of energy storage may not have been a primary factor in Winter Storm Uri’s impact, it should not diminish the fact that Texas currently has 35% intermittent resources in its entire generation mix (i.e., wind and solar, to achieve low variable costs and zero emissions), which cannot be dispatched precisely when needed.[16]
A June 11, 2021, article by the American Bar Association (ABA) stated, “All generating resources are not equal in function or value from a system reliability standpoint. Dispatchable resources are the most valuable from a system integrity and operational efficiency perspective as dispatchable resources are available when demanded, and electricity must be generated at the moment it’s consumed.”[17] In that same article, the ABA stated, “Texas adopted an energy-only market structure years ago during deregulation of the electric markets. While theoretically viable, an energy-only market conceals flaws induced by regulatory/political externalities and technology advancements, which are usually only exposed through extreme circumstances.”[18]
In March 2021, electricity generated in Texas was 40% from natural gas, 14.5% from coal, 10% from nuclear, 0.5% from hydropower, and 35% from non-hydro renewables, mainly wind and solar power. Texas has more wind capacity than any other state but still retains more than 54% carbon-based generation. The pressure from regulators and investors continues to direct future electrical generation growth and replacement needs with non-carbon-based, intermittent resources; as such, the trend would appear to be an expansion of investment in wind and solar generation capacity.
Though carbon-free, both wind and solar are primarily intermittent, non-dispatchable forms of electricity generation with capacity factors at a half to a two-thirds less than current dispatchable carbon-based resources. Figure 3 reports current and future energy storage —primarily in the form of batteries—planned for Texas to attempt to offset the intermittency of the growing generation resource mix. Though energy storage may somewhat offset, some of the issues related to non-dispatchable generation resources, duration of discharge—especially for battery resources—is somewhat limited both in terms of economics and technology. As of October 2021, about 1100 MW of grid-connected battery storage in service has been placed in service, with an additional 4,000 MW planned by March 2023.[19]
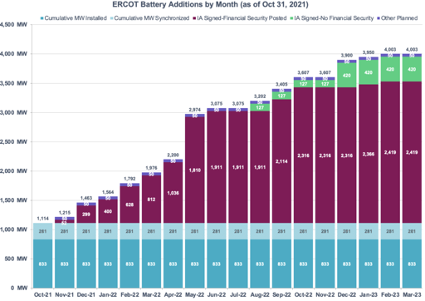
Figure 3 ERCOT Battery Additions by Month (as of October 31, 2021)[20]
In terms of conventional dispatchable, base-load generation that is carbon-free, nuclear energy is the only current resource in the market. Even though nuclear energy is carbon-free, dispatchable, and has capacity factors exceeding 95%, its expansion has been hindered by significant capital cost overruns, concerns for fuel disposal, and overall perceived issues related to operational safety such as highly publicized global disasters at Chernobyl and Fukushima. There is no current plan to significantly expand the nuclear fleet in the U.S.
Another potential form of reliable, carbon-neutral or carbon-free electrical generation currently being evaluated is in the form of hydrogen as a fuel. Reliability would be on par with other forms of base load generation. A significant amount of emphasis is being placed on this technology since it would be both dispatchable and continuous as long as hydrogen fuel is available. There are essentially three forms of hydrogen currently being investigated: gray, blue, and green. Gray hydrogen is created from fossil fuels, and the process releases carbon dioxide which is not captured.[21] Approximately 98% of current hydrogen production is gray and comes from steam reforming and gasification and produces equivalent (if not more) CO2 than other forms of carbon-based fuels.
Blue hydrogen uses the same process as gray, except the carbon is captured and stored in the process. In concept, 90% of the CO2 can be sequestered and stored but the challenge is availability of long-term storage. This makes it much more environmentally friendly but comes with added technical challenges and a big increase in cost.[22] Green hydrogen is a net-zero carbon fuel wherein all our electricity and fuel are produced by emission-free sources, i.e., renewables. To produce green hydrogen through electrolysis requires a significant amount of electricity, which means a staggering increase in the amount of wind and solar power to meet global targets. Some current estimates project that more offshore wind capacity than in the previous 20 years—every year for the next 30 years—would need to be installed to meet capacity. Finally, costs are significant at this point in time.
Conclusion: Quality Versus Quantity, and Effects on Business Decisions
The debate continues as to the reasons for the significant impacts and business interruptions associated with events like Winter Storm Uri. What has become apparent in the aftermath of that storm is that many have yet to fully understand or appreciate the significant changes occurring in the U.S. bulk electrical energy market. The example used in this paper, though rather unique due to the energy-only market configuration of Texas, is a bellwether for the country. Regardless of the causes of weather events challenging electricity grids, a steady transition in the U.S. from carbon-based energy resources to one that is either less or completely carbon-free, and the difference in quality and quantity of available energy associated with that conversion, will persist. While there is some disagreement as to whether the challenges encountered during natural extreme weather events like Storm Uri have been amplified by the current energy transition process, it is apparent that energy storage is a key component of the transition process and, ultimately, achieving carbon neutrality or net-zero carbon emissions.
Perhaps the greatest challenge of energy transition is ensuring new and/or developing forms of energy storage can support and maintain the current system reliability requirements to which the U.S. population has become accustomed, at an affordable price. Any negative impacts related to pricing of energy will ultimately be carried by the consumer, but intermediate cost risks for the businesses associated with project capital as well as insurance, underwriting, and other risk management related issues may also be negatively affected. Will an event like Winter Storm Uri impact the U.S. market again? Absolutely. Could it produce similar results in terms of human calamity and business impacts? Most likely. Risk managers should carefully consider the potential challenges to electrical service reliability and increased potential for business interruptions as we advance through this period of energy transition.
[1] JD Taft, PhD, Electric Grid Resilience and Reliability for Grid Architecture (November 2017).
[4] Net generation for all sectors, Texas, Fuel Type-Check all, Annual, 2001–20". www.eia.gov.
[6] Hughes, N, Agnolucci P. 4.03 - Hydrogen Economics and Policy, Editor(s): Ali Sayigh, Comprehensive Renewable Energy, Elsevier, 2012, ages 65-95, https://doi.org/10.1016/B978-0-08-087872-0.00417-0. (https://www.sciencedirect.com/science/article/pii/B9780080878720004170)
[8] J.W. Busby, Et Al., Cascading risks: Understanding the 2021 winter blackout in Texas, Energy Research & Social Science, Volume 77, July 2021, 102106.
[9] The February 2021 Cold Weather Outages in Texas and the South Central United States | FERC, NERC and Regional Entity Staff Report, November 16, 2021.
[14] Witt, Adam & Chalise, Dol Raj & Hadjerioua, Boualem & Bishop, Norm & Manwaring, Michael. (2016). Development and Implications of a Predictive Cost Methodology for Modular Pumped Storage Hydropower (m-PSH) Projects in the United States. 10.13140/RG.2.2.10441.54881.