[authors: Sharkey Bowers, Scott Hollingsworth, and Richard Stahl]
Introduction
Geotechnical practitioners are often focused on the “Three G’s”—namely geology, groundwater, and geometry. The interconnectedness of the Three G’s is responsible for fundamental aspects of the Earth’s composition and hydrological processes. Understanding the relationships between the Three G’s is crucial for various fields, including environmental science, engineering and infrastructure development, and resource management. From historically influencing where and how human settlements develop to controlling the engineering design and construction of new developments, failure to respect the Three G’s can occur at peril.
The foundations of all human construction and developments rest upon the earth beneath our feet. The Geology, behavior of groundwater, and the geometry of the surface and subsurface conditions must be understood before humankind embarks to build upon, build through, excavate within, retain, or support the ground. Geologic conditions will control how we design and build foundations, the machinery needed to excavate for construction, and in some instances, the locations that we decide to develop. Likewise, the geometry of both the landscape and subsurface soil and rock layers will have considerable influence on the design and cost to develop a site. Finally, understanding the groundwater conditions is essential whether the intent is to develop a source of water supply, prevent pollution and protect environmental systems, or to effectively design and construct buildings and/or infrastructure.
In this article, we discuss examples of how the Three G’s have dictated human development; creative solutions that show how the Three G’s can be used for human benefit; and, to the contrary, how the Three G’s can cause multi-million-dollar property damage, delays on construction sites, and/or injury and loss of life if they are not adequately considered. The following information may be of particular interest to property owners, developers, engineers, architects, property insurance professionals, and attorneys working in the field of construction defects.
Venice Lagoon – A Case Study in Human Adaptation to Geologic Conditions
The lagoon of Venice, Italy is one example of how the Three G’s have shaped the world we live in today. The present Venice lagoon, interspersed with channels and canals that are used as a primary mode of transportation, is mainly the result of human development following glacial retreat and resulting coastline development some 6,000 to 7,000 years ago. Dating back to Ancient Rome, the Venetians understood early on the strategic importance of the lagoon. Surrounded by water, it served as a natural barrier from invading enemies and a hub for maritime trade.
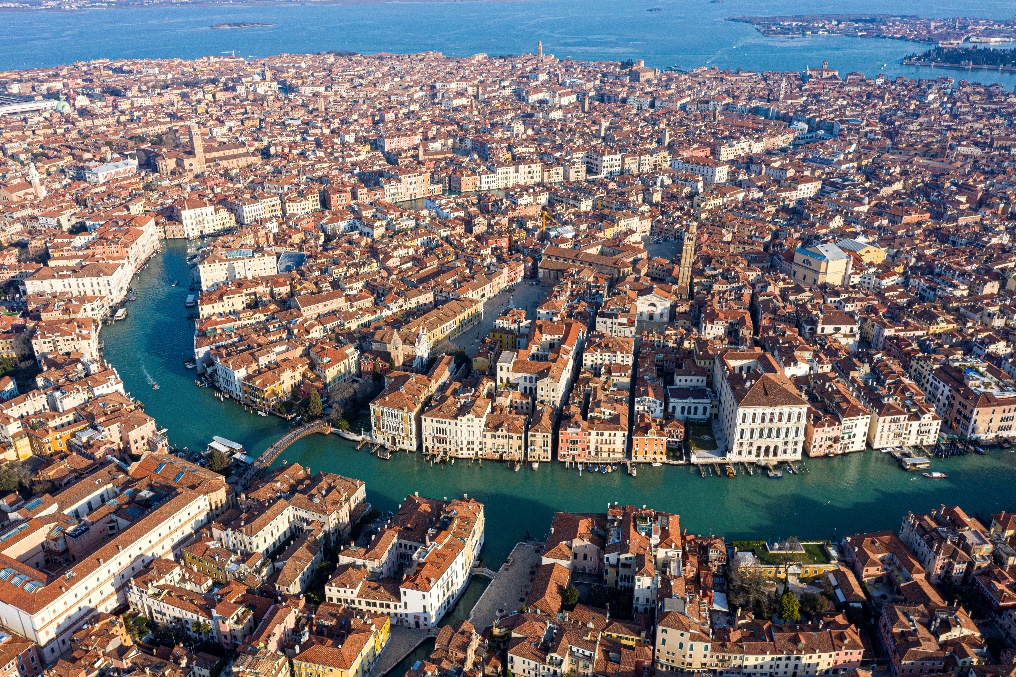
Figure 1 - Aerial view of modern-day Venice.
However, the geology of the area presented a challenge to construction and development. The area was primarily a wetland comprised of mud flats, salt marshes, and tidal shallows with a minority portion of the lagoon covered by open water or dry land. The shallow soils in the area consisted primarily of peat, which is an organic material that is not suitable for foundation support, due to its high compressibility or settlement under load and potential to decay with time. To prevent damage to their buildings, the Venetians developed a method to install deep foundations that are similar to modern-day timber piles. They would harvest tall trees from nearby forests and drive them down into the ground to the depth of the hard clay layer that underlies the organic peat. The hard clay layer would help support the piles through end bearing and frictional resistance. Horizontal layers of timbers were laid on top of the vertical piles, then limestone was placed on top of the timbers to create a building pad.
There are a variety of soils that remain a challenge for engineers and contractors alike, and organic soils are no exception. Failure to identify organic soils before construction can result in cost increases, often with both the design and schedule experiencing change. If organic soils are not identified until after construction is complete and settlement-induced damage has occurred, then repair costs and schedule impacts will dominate the project. A thorough geotechnical investigation program, coupled with the development of a geotechnical baseline or model, is essential to prevent these types of issues from detrimentally affecting a project. The cost of a comprehensive geotechnical investigation and baseline model development is inexpensive when compared to the cost to remediate these types of issues during or after construction.
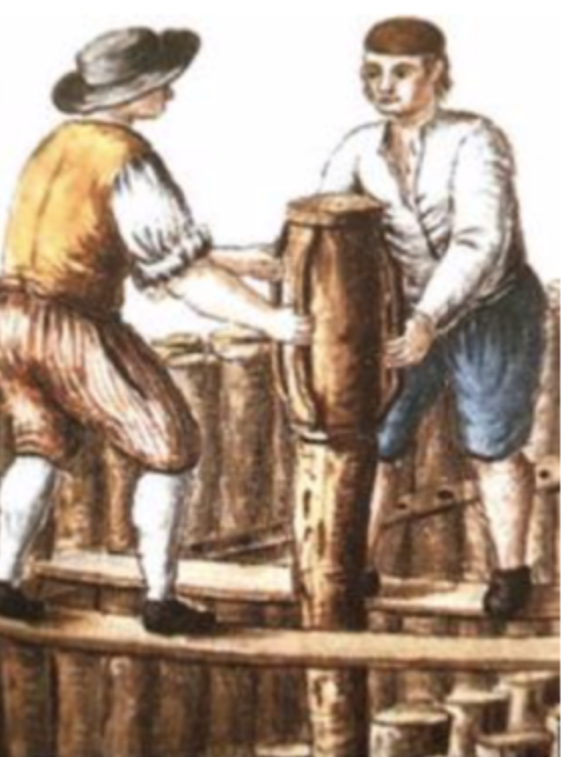
Figure 2 – Historical depiction showing timber pile installation for foundations in poor shallow soil conditions (Source: https://theconstructor.org/case-study/venice-foundation-details/224185/).
Sinkhole Formation and Remediation
Organic soils are only one of many geologic conditions that can cause complications for construction. Sinkholes are another geological feature that commonly can cause expensive settlement-related damage to buildings, or costly construction delays. They are prevalent in areas that have carbonate bedrock such as limestone and dolostone. Sinkholes develop through groundwater migration and dissolution of the bedrock over time. The dissolution causes the formation of voids, which commonly remain undiscovered if the overlying soil has sufficient strength and thickness to bridge over the void. However, if the void in the bedrock is sufficiently large, and/or the thickness of overlying soils is relatively thin, sinkholes develop as the overburden soils ravel or collapse into the void; this can cause depressions or holes in the ground surface. Structures that rely upon the underlying soil or rock for support can be impacted.
Sinkholes can cause damage to existing residential, commercial, and industrial buildings, and have also been known to cause damage or significant delays on construction sites. Often, development can trigger sinkhole activity due to the concentration of water in an isolated area, such as a stormwater pond or underground water detention facility, or due to modifications to the groundwater table due to dewatering. Proper investigations and planning before construction can limit the potential for sinkholes to detrimentally affect a construction project.
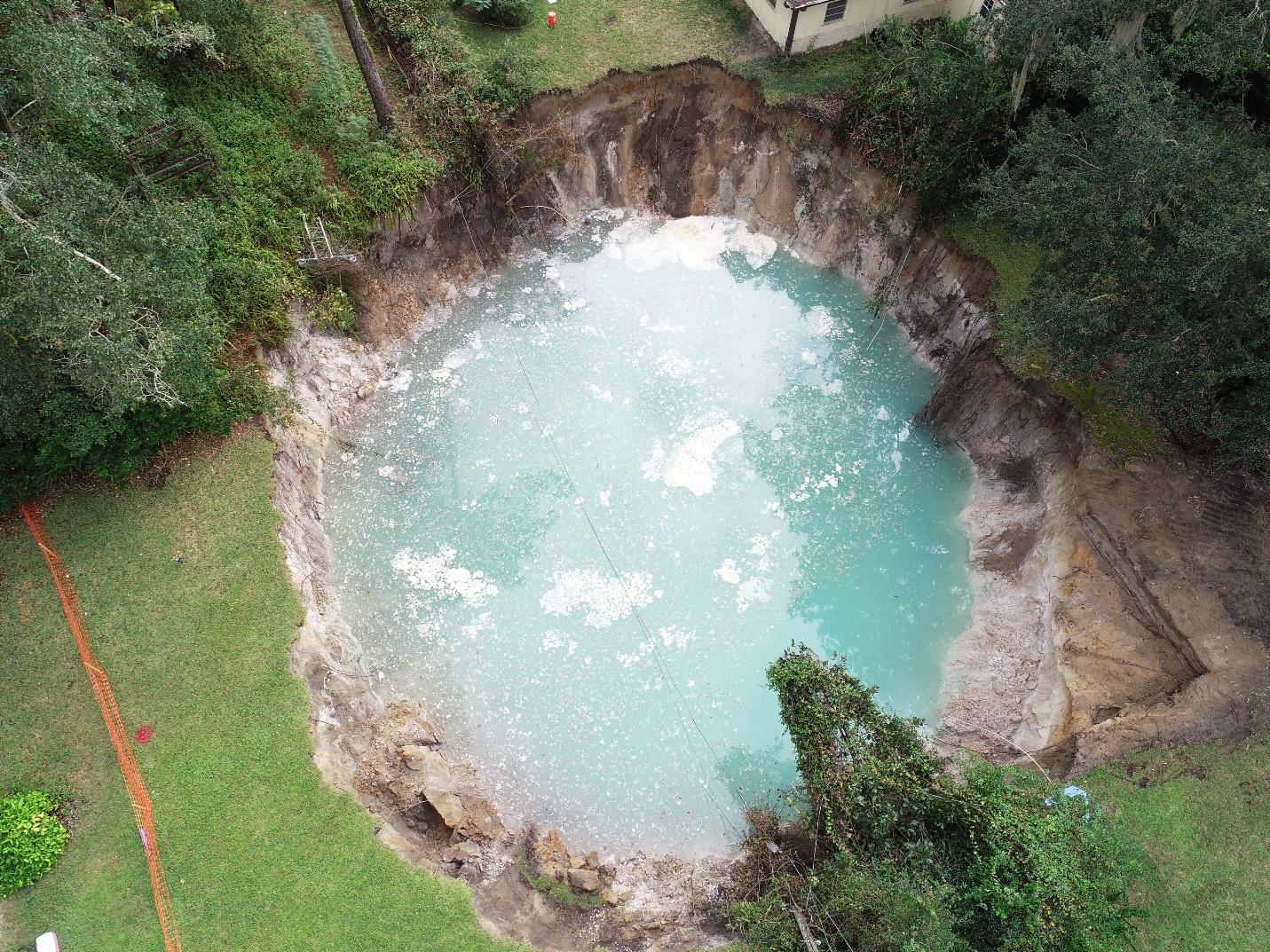
Figure 3 - Overview photograph of a sinkhole that developed near a residence in Central Florida.
Remediation of sinkholes can be achieved via grouting or an inverted rock filter, whereby the ground is excavated down to the depth of bedrock then backfilled with boulders, cobbles, and gravel, in a carefully engineered sequence with geosynthetic filter fabric to bridge over remaining voids, avoiding migration of soils due to gravity and groundwater. This type of remediation can only be performed if the sinkhole is identified before construction.
Post-construction settlement damage to buildings caused by sinkholes is typically addressed by grouting and/or underpin installations. Grouting involves installing steel casing to the depth of bedrock and injecting compaction grout in the overlying soils as the casing is withdrawn to improve any raveled or loosened soils. Underpinning involves the direct transfer of the structural loads to the competent bedrock. It typically involves the installation of small-diameter steel piles that are drilled or pushed into the ground then attached to foundations of the building.
Los Angeles Basin – Historical Development & Groundwater Conditions
Los Angeles, California, settled in the late 1700s, serves as another historical development that was shaped by the geometry of the natural landscape as well as geology and groundwater conditions. Because it is in a semi-arid coastal plain, rainwater could not be relied upon to consistently supply fresh water. The Los Angeles River was known for flooding during periods of heavy rain, but precipitation would reduce to a mere trickle during other times of the year. To meet fresh water needs, early settlers turned their attention underground. Farmers discovered multiple natural aquifers by drilling wells from which a seemingly endless supply of fresh water could be obtained.
By drilling wells into the ground, farmers were able to access fresh water from aquifers (underground layers of permeable rock or soil which contain or transmit water). Wells drilled down into the sand and bedrock aquifers would produce artesian conditions, where the groundwater is under sufficient pressure—fed by geometry or elevation differences—to cause the water to exit the wells without the need for pumping. The subsurface water pressure forces water up through the ground surface when the confining layer is breached, such as by a well. See Figure 4 for an illustration of an artesian well.
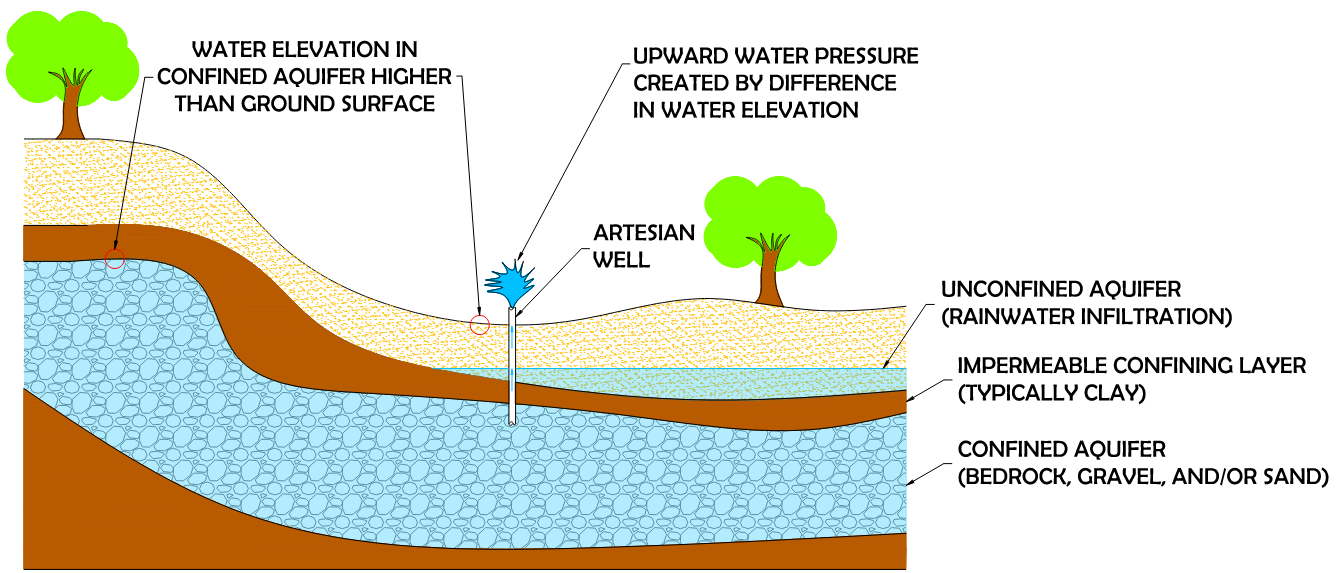
Figure 4 - Graphic depicting artesian conditions. Note that the groundwater level in the confined aquifer is higher than ground level at the well.
While artesian conditions were beneficial for the early farmers in Los Angeles, as well as many other locations throughout the world, these conditions can create challenges during design and construction of subsurface features, and potentially significant damages if not identified and accounted for in advance. Piping and erosion of soil, slope or braced or tied back excavation failure, and disturbance of more competent soils include some of the mechanisms that can transpire if artesian conditions are not properly considered in advance of design, tendering, or construction – not to mention the additional dewatering costs that will be required to prevent a flooded construction site. Investing in a comprehensive site investigation program, including geotechnical and hydrogeological studies, can help avoid significant increases in costs of construction and other ancillary costs including business interruption, legal, and expert costs that may result if artesian conditions are not identified before construction begins.
Dewatering in Construction
When constructing foundations or utilities below the groundwater table, contractors are commonly required to dewater the site to construct in dry conditions. Dewatering involves drawing down the water table with the use of pumps. If only limited dewatering is required, a sump pit can be excavated, and a pump can be placed in the sump pit to pump the water out. With the pump running, water will flow from the surrounding area through the soil into the sump pit, or the water can be diverted to the sump pit via berms and ditches. If deeper dewatering is necessary, dewatering wells are usually required to lower the water table.
If the construction site being dewatered has homogenous sandy or gravelly soil conditions, dewatering can be relatively straightforward with some exceptions. Sands and gravels have larger connected void spaces than silts and clays and can be dewatered through pumping in a shorter period. The challenge with such soils is the volume and flow rate of pumping required to achieve effective dewatering and depressurization. It is often more challenging to dewater finer-grained soils such as silts and clays, or silts and clays that are sequenced between sand and gravels, with greater time required to achieve effective dewatering. In these types of soil deposits, the average groundwater flow rate in the vertical direction will be as slow as the slowest-draining soil. In the horizontal direction, the groundwater, on average, will flow as fast as the fastest draining soil. This is because low-permeable soil layers such as clay will function as a confining layer, slowing or prohibiting the downward flow of water. The groundwater will “perch” and flow laterally on top of the silt and clay layer. In all cases, dewatering a construction site can also influence the water table off‑site. Consideration needs to be applied to third party facilities which may rely upon the groundwater for water supply, or which may be negatively impacted through dewatering-induced soil consolidation and settlement.
Los Angeles Aqueduct – A Case Study in the Use of Landscape Geometry to Human Advantage
By the early 1900s, groundwater from the rivers and aquifers alone was not sufficient to supply the growing population of Los Angeles. Fresh water became a scarce commodity, and the city engineer developed a plan to construct an aqueduct that provided fresh water from Owens River, located 233 miles away in the Sierra Nevada Mountains. The aqueduct consists of a series of dams, reservoirs, siphons, tunnels, canals, and conduit. The topographic relief across the aqueduct is approximately 3,500 feet, meaning that the aqueduct relies on ground topography and gravity, allowing for the water to flow downhill from the mountains to Los Angeles. It is this same topographical relief that helped pressurize or feed the aquifer and artesian wells as discussed above.
Constructing The Los Angeles Aqueduct is considered by many to be one of the greatest engineering feats of our time. It is a robust, yet simple system that relies on the geometry of the surrounding landscape to provide fresh water supply from hundreds of miles away to the city.
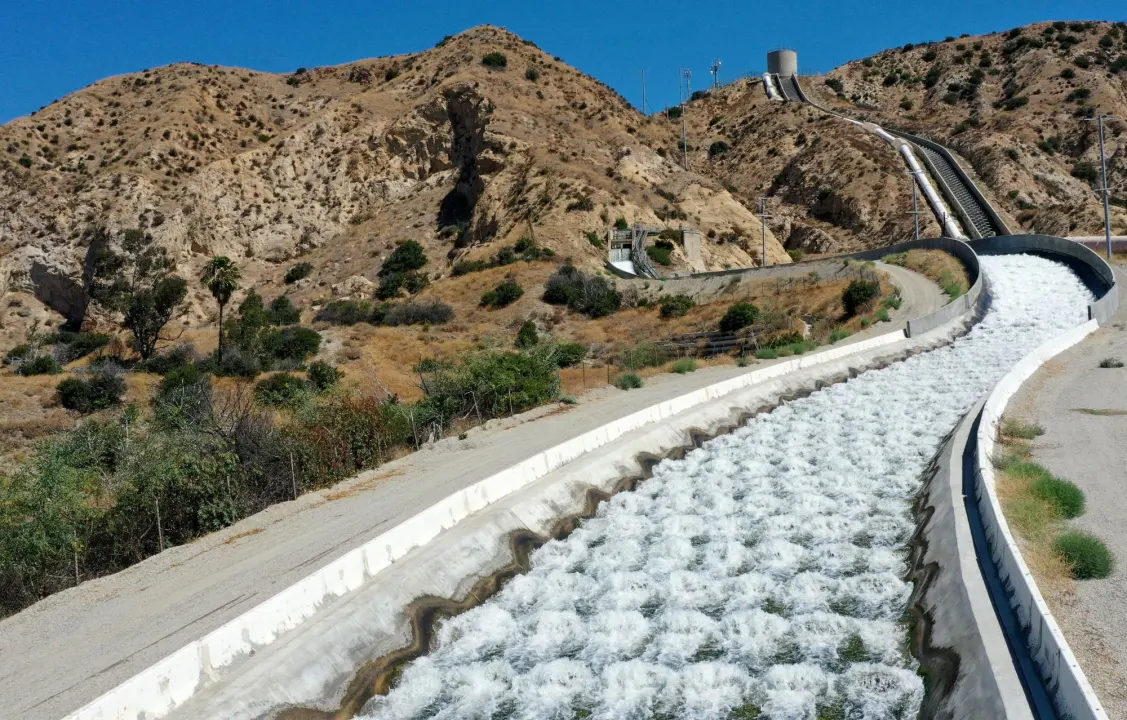
Figure 5 - Water flows down the Los Angeles Aqueduct, relying on the geometry of the landscape to gravity flow down toward the City of Los Angeles (Photo by Dean Musgrove, Los Angeles Daily News/SCNG; Source: https://www.dailynews.com/2022/11/04/proposal-to-place-solar-panels-over-la-aqueduct-advances//).
While the Los Angeles Aqueduct is an example of using the geometry of the ground surface to the advantage of human development, slopes and hilly terrains can also provide challenges that must be properly accounted for to successfully develop these types of landscapes.
Slopes in Hilly Terrain
Finally, along with geology and groundwater, site geometry plays a significant role in urban development. Although there may be aesthetic benefits in constructing on sloping ground versus flat ground, the challenges and costs can be greater. A good understanding of the foundations is required to ensure that the soils can provide an adequate bearing capacity. Depending upon the size of the development and slope angle of the ground, cut and fill slopes may be required. Developments may also be at the top or bottom of slopes, which introduces certain risks. There are many towns and cities across the globe where constructing on or nearby steep, hilly terrain is the norm, with Hong Kong being one of the most famous examples.
Rapid population growth and substantial economic expansion in Hong Kong since the 1960s have been accompanied by extensive civil engineering and building works. To house the now approximately 7.4 million people within an approximately 680 square miles (1100 km2) land mass that has over 140 mountains or peaks that rise over 980 feet (300 m) above sea level, with ten (10) over 2,300 feet (700 m) above sea level, a considerable number of man-made slopes and retaining walls were required over very hilly territory. However, dating back to the 1960s, there was limited geotechnical control of slope design and permitting, resulting in questionable construction and stability of many of these slopes and retaining walls. The vulnerabilities of these slopes and retaining walls became exposed through increasing development, steepening of existing terrain, and severe rainstorms.
As development and population increased, landslides and fatalities were taking place at increasing levels. Catastrophic landslides in 1972 and 1976 as portrayed in Figure 6 ultimately led to the development of the government’s Geotechnical Control Office (GCO) in 1977, which was renamed in 1991 to the Geotechnical Engineering Office (GEO) (Wong, 2017).
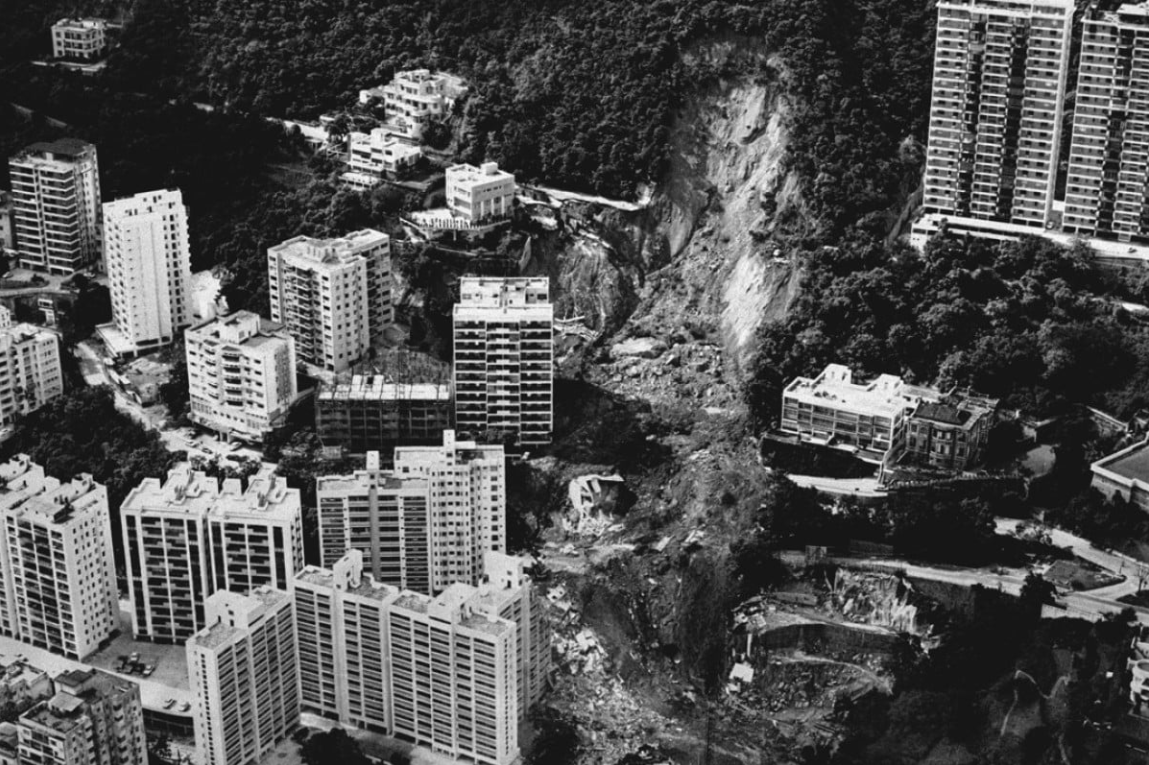
Figure 6 - 1972 Po Shan Road Landslide in Mid-Levels of Hong Kong that toppled a 12-story building and caused 67 fatalities (Source: https://www.scmp.com/magazines/post-magazine/short-reads/article/2098525/deadly-1972-twin-landslides-hong-kong-claimed).
Figure 7 shows how the number of landslide failures and associated fatalities increased then lessened following establishment of the GEO. The decrease in landslide fatalities is attributed to the introduction and establishment of a more rigorous approach to cataloging existing man-made slopes and retaining walls (now about 60,000 within the region), conducting thorough investigations to characterize the soils, carrying out groundwater assessment, and then either remediating existing slopes, or designing and constructing new slopes, using robust designs.
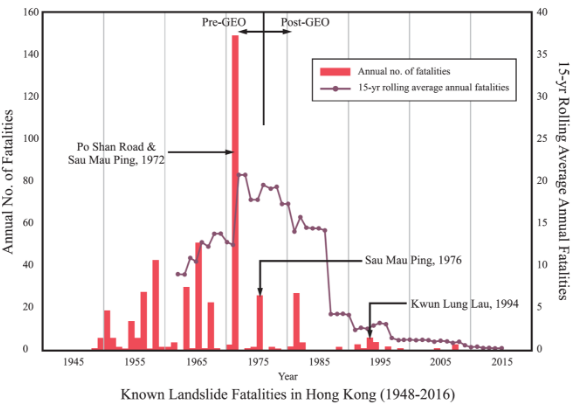
Figure 7 - History of landslide fatalities in Hong Kong from 1948 to 2016 (Morgenstern 2021)
These implementations and others, including the development of quantitative risk assessment to slopes that also factor in climate change and changing (increasing) precipitation loads in Hong Kong, have all led to the near elimination of fatalities due to landslides in Hong Kong. The authors have had the opportunity to investigate, design, and construct slope stabilization projects in Hong Kong, as well as investigate slope instability defense barriers as part of a QRA initiative, in situations where natural slopes extend tens to hundreds of meters upslope from a development.
Conclusion
The interplay of the Three G’s has shaped the world that we live in today and has unavoidable impacts on human construction activities occurring all over the globe. Understanding the relationships between geology, groundwater, and geometry is crucial when undertaking projects both new and old. It is particularly important to bear these factors in mind to avoid unnecessary and costly complications during or after the construction of buildings. When taking on new projects, especially those in areas with complex ground soil/rock formations, unusual or unpredictable water tables, challenging slopes, and so on, stakeholders should consider enlisting the help of expert engineers and geologists who can provide relevant, valuable insight and guidance.
Acknowledgments
We would like to thank W. Sharkey Bowers, PE, Scott Hollingsworth, PG, and Richard Stahl for providing insights and expertise that greatly assisted in this research.
References
- Brambati, A., Carbognin, L., Quaia, T., Teatini, P., and Tosi, L. “The Lagoon of Venice: geological setting, evolution and land subsidence.” International Union of Geological Sciences. September 2003.
- “William Mulholland Biography” webpage. Water and Power Associates website. https://waterandpower.org/ museum/Mulholland_Biography.html.
- “Water in Early Los Angles” webpage. Water and Power Associates website. https://waterandpower.org/ museum/Water_in_Early_Los_Angeles.html.
- “The Los Angeles River – The Unpredictable!” webpage. Water and Power Associates website. https://waterandpower.org/ museum/Los_Angeles_River_The_Unpredictable!.html.
- Peck, Ralph B, Hanson, Walter E., and Thornburn, Thomas H. “Foundation Engineering, Second Edition.” John Wiley & Sons. 1974.
- Morgenstern, N.R., 2021. “Geotechnical Risk, Regulation, and Public Policy,” The Sixth Victor de Mello Lecture, 2021, 48 pages
- Wong, H.N, “Forty Years of Slope Engineering in Hong Kong,” The HKI Geotechnical Divisional Seminar 2017, 10 pages.